Sponsored Content by MGIApr 13 2023
Skip to:
With the 7th birthday of MGI this April coinciding with the 20th anniversary of the Human Genome Project, we look back at where it all started as well as the importance of sequencing technology in advancing the field. In this inspiring interview, we speak to Dr. Radoje Drmanac, co-founder of Complete Genomics and Chief Scientific Officer at MGI, to learn more.
The Human Genome Project (HGP) is celebrating its 20th anniversary this April. As someone involved in this project from the start, how did this project come about, and what was your involvement?
The field of genomics was already around before the Human Genome Project started in the 1980s. Many people like George Church, Hans Lehrach and myself were involved in thinking about how to create chromosome maps or expression sequence tags. Through such thinking, I became involved in genetic engineering at the Institute for Molecular Genetics and Genetic Engineering in Serbia. There I started thinking about how to improve DNA sequencing as sequencing at that time was carried out using the Sanger method. Although I never used this method myself, I watched my colleagues conduct gel sequencing, and it soon became clear how slow and difficult it was.
In 1987, I proposed a new method for more efficient sequencing based on DNA arrays called sequencing by hybridization (SBH). At the same time, the US Department of Energy (DOE), developer of atomic bombs, was studying the impact of radiation in causing genetic mutations. They held a meeting to discuss how to find and identify these mutations, and I believe George Church told them that the best way would be to sequence the whole genome in exposed people. They initiated a first round of grants to improve technologies for genome analysis, including sequencing. I applied for this grant from Serbia in 1987, and to everybody’s surprise, including my own, we received a $150,000 grant to develop that sequencing by hybridization method that held so much promise for faster, much higher throughput sequencing.
In 1988, I proposed massively parallel sequencing (MPS) using microarrays, a radically new approach, and the proposal was to use emulsion polymerase chain reaction (PCR) on micro-sized beads to create millions of clonal DNA on such small beads to form these novel DNA microarrays. Before this, picking and arraying clones was the only viable method.
Then, as a postdoc in 1991, through this connection with the DOE, the whole team – all nine of us – moved to Argonne National Laboratory near Chicago in the US to continue to develop sequencing by hybridization technology using the DOE grants.
We also published a couple of papers, the first being in journal Genomics, a paper on genome sequencing by SBH that was very popular. Then in Argonne National Laboratory, together with Lee Hood, we published a paper in the journal Science in 1993 that proved in a blind test that sequencing by hybridization was highly accurate and could be used to sequence DNA using oligo hybridization.
That is how I first became involved in the Human Genome Project.

Image Credit: Elena Sharipova/Shutterstock.com
How did the Human Genome Project help to propel parallel sequencing technologies in the life sciences?
I proposed massively parallel sequencing in 1988, and sequencing by synthesis was also proposed in the 1990s, both long before the genome was sequenced. However, once the genome was sequenced, these two technologies and our sequencing by ligation became possible, allowing for routine, affordable individual genome sequencing.
This motivated me to start Complete Genomics in 2005, acquiring funding and starting operations in 2006. In 2005, we invented DNA nano balls and pattern arrays for massively parallel sequencing. These clonal arrays were critical to implementing efficient massively parallel sequencing, and our DNA nano balls were, and still are, the best approach for this. We were the first to implement pattern arrays, while others used random arrays.
When we started Complete Genomics, within a couple of years of implementation, we achieved a milestone, an event that promoted the genomic revolution. We sequenced and published in the journal Science in 2010, for the first time, a whole human genome for $5,000, a 10-fold improvement, using patterned DNA arrays of DNA nanoballs, a much more efficient, higher-density sequencing platform.
Seven years after the first genome was sequenced for $3 billion, your team published a $5000 genome, almost 1 million fold cheaper. What was this journey like, and were there any particular R&D challenges that you had to overcome?
Many people in the industry, including some of the people we hired, believed that such dense arrays, were unachievable. Despite this initial resistance, we started making the arrays, and the first genome was sequenced with, I believe, 600 nanometers center to center, a sub-one-micron array. We have since continued to push the edge of possibilities. One limitation at the time was that cameras had fewer pixels than they do today. Today, we have cameras with a hundred times more pixels and much faster imaging.
As the industry was still new, we had to offer it as a service initially. As such, we started this service for routine genome sequencing for scientists. They would send us their important samples, and we would sequence them. Nonetheless, there was still a lot of debate regarding the accuracy of whole genome sequencing by short reads and, despite our efforts, we still had some errors, so even though it was quite accurate, it was not yet perfect.
There were also questions surrounding whether we could detect a single mutation that causes a disease. As this had never been carried out before, and as the genome is so big comprising 3 billion chemical letters, there were also uncertainties regarding our ability to identify the single mutation that causes disease.
All things considered, it was really rewarding when, with a long-time friend and colleague, Lee Hood, we decided to sequence the first family. We sequenced a family of four, two parents and their two children who were affected with a disease called Miller Syndrome, to prove that we could identify the cause of Miller Syndrome in the affected children.
We successfully detected the genetic cause of this disease and published our findings that demonstrated our ability to sequence genomes accurately. These results also highlighted the importance of sequencing family genomes and their role in discovering genetic diseases. With that paper and the many others that followed, people soon realized that the genome could be sequenced efficiently, affordably, and quite accurately, and this is what kickstarted the genome revolution.
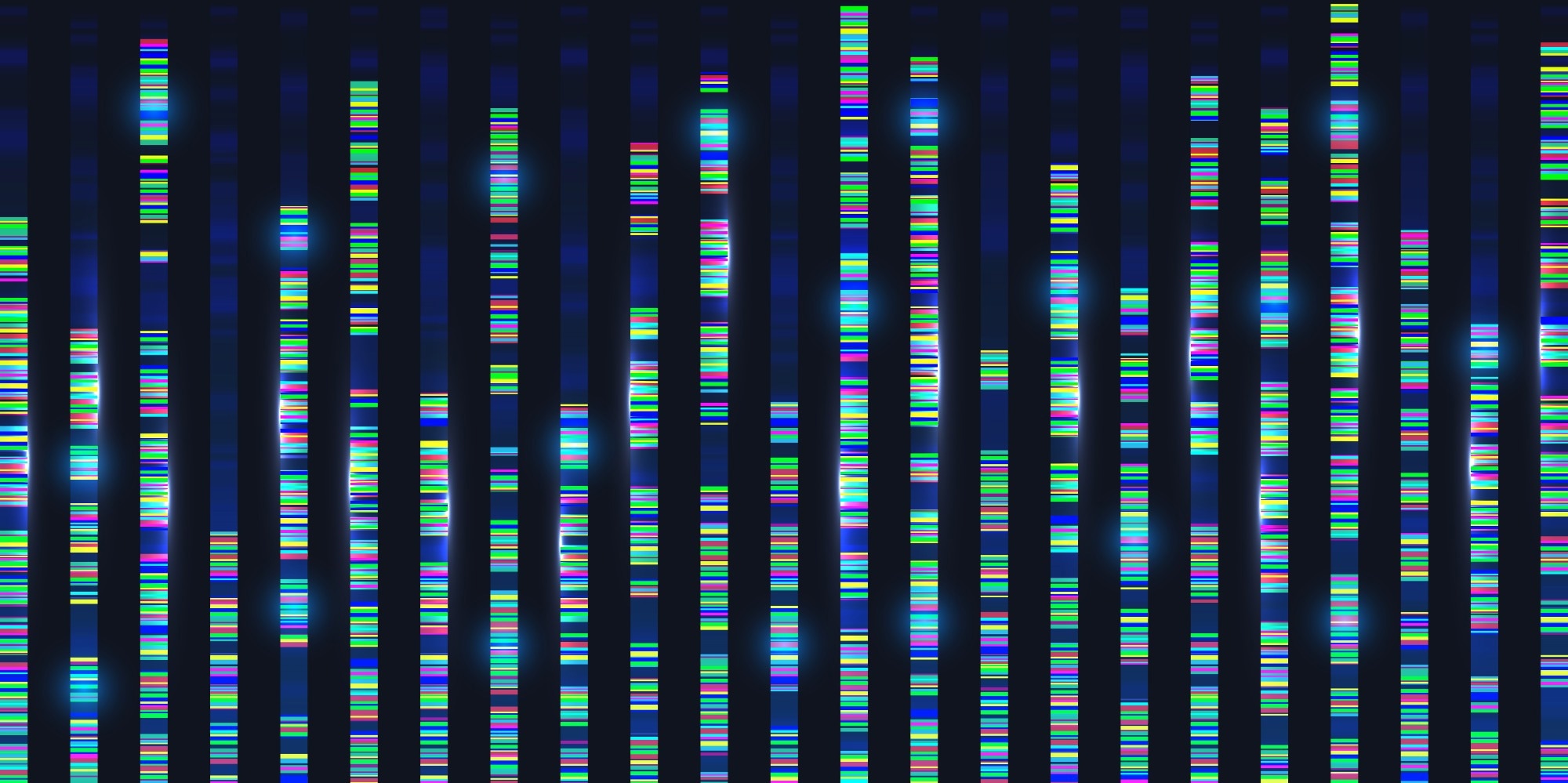
Image Credit: MIKHAIL GRACHIKOV/Shutterstock.com
You have had an incredible career, from being the technology developer for the Human Genome Project to the sub-hundred dollar human genome and CoolMPS technology. Over the past 20 years, how do you feel the genomics industry has evolved?
In one sentence, the genomic revolution was made possible by massively parallel sequencing. Everybody knows that the progress we continue to see would not be possible without this technology. The cost would not have been able to come down from $5,000 to $1,000 or even to the very first $100 and sub $100 genome. Over the last 20 years, many applications have been developed for sequencing because of this ability to carry out efficient sequencing in almost any lab. Today, almost every aspect of omics uses sequencing.
We measure protein levels in the blood by sequencing barcodes. People measure gene activities by sequencing. Sequencing became the single assay to measure the molecular state of our cells. It was not just genome sequencing, but all other omics were enabled by MPS. At the same time, long single-molecule sequencing was also achieved and made available by multiple companies.
The revolution then spilled out into knowledge by sequencing all of these samples, having all these assays, sequencing millions of genomes. We also learned how to barcode single cells, and now, using dens DNA nanoball arrays, we have the best spatial omics to monitor gene expression in tissue sections at the subcellular level. All this is enabled by massively parallel sequencing, and these applications are still growing.
We have seen in recent years that sequencing is the key to accelerating the field of omics. What role have both Complete Genomics and MGI played in this discovery, and what role will they continue to play in its future?
We have always pushed the boundaries, starting with the $5,000 genome, which was so important. In a way, it proved that routine genome sequencing was possible and that there is value in individual genome sequencing. After this, we continued to build on initial studies and develop many different instruments.
Our DNBSEQ-T7*will be remembered as a critical instrument, the first to achieve pair-end 2x150 bases high-throughput genomes in 24 hours. The T7* is an example of the power of DNBSEQ when it is properly implemented with really cool engineering.
We have now also announced DNBSEQ-T20×2RS*providing a sub-hundred-dollar genome. Our DNBSEQ provides the highest resolution spatial omics due to the high-density arrays. I believe we will be the first to achieve the $10 genome, as there is no doubt that DNBSEQ enables that. We can make four times higher array density than today. The drive for the $10 genome is not just for the inherited genome but because it will allow annual checkups using deep sequencing in the immune system, cell-free DNA, and in the microbiome, enabling us to monitor our health and our aging at the molecular level.
One technology that is a less expensive and more efficient technology for sequencing is CoolMPS, as it allows much longer reads in massively parallel sequencing. We have already implemented SE400 using standard sequencing technology. With CoolMPS, we are working on getting to 1,000 bases continuous reads, the SE1000 – continuous MPS for 1,000 bases. This is so important because it will bring affordable genome sequencing to an even higher level of completion. There are some types of sequences, like triple repeats, that, when they expand, cause a disease like fragile X. With these longer reads, we will be able to measure how long these repeats are, which will tell us if expansion is causing disease or if it is still tolerable.
Combining the efficient sequencing with our single tube LFR technology for haplotype phasing, solving blind spots by resolving the pseudogenes, separating pseudogenes from the real genes, and using these longer reads all redefines massively parallel sequencing and brings it to the level that will enable faster understanding of our genetic program. With this understanding, our omics tests will be so efficient that we can bring health monitoring omics to all.
We will have such a deep understanding that when we obtain the state-of-the-art measures of our tissues at the molecular level, we will know what this means and how to prevent disease and slow aging. We already have a level of understanding that our tissues are primarily aging because of epigenetics. In a way, epigenetics is controlled by a genome program that uses methylation and other marks to differentiate cells. In the beginning, there is only one cell, and then we have hundreds of tissues in the developed organism. Every tissue is locked into its state by these methylation and other marks called epigenetic modifications. These marks are lost or changed during life, i.e., modified, leading to a loss of young and healthy tissues. We can reverse this process using transcription factors that promote the re-establishment of young tissue epigenetic marks. However, it is important that we do not just live longer but that we have longer healthy and more productive lives.
Everything in biosciences is growing exponentially thanks to these technologies. Our understanding is exponential, and our technology’s ability to measure things is exponential. We will definitely have a $10 genome within this decade, and 1,000 base MPS reads, so that, in a decade or two, after deep understanding of our molecular nature maybe we will be in control of aging.
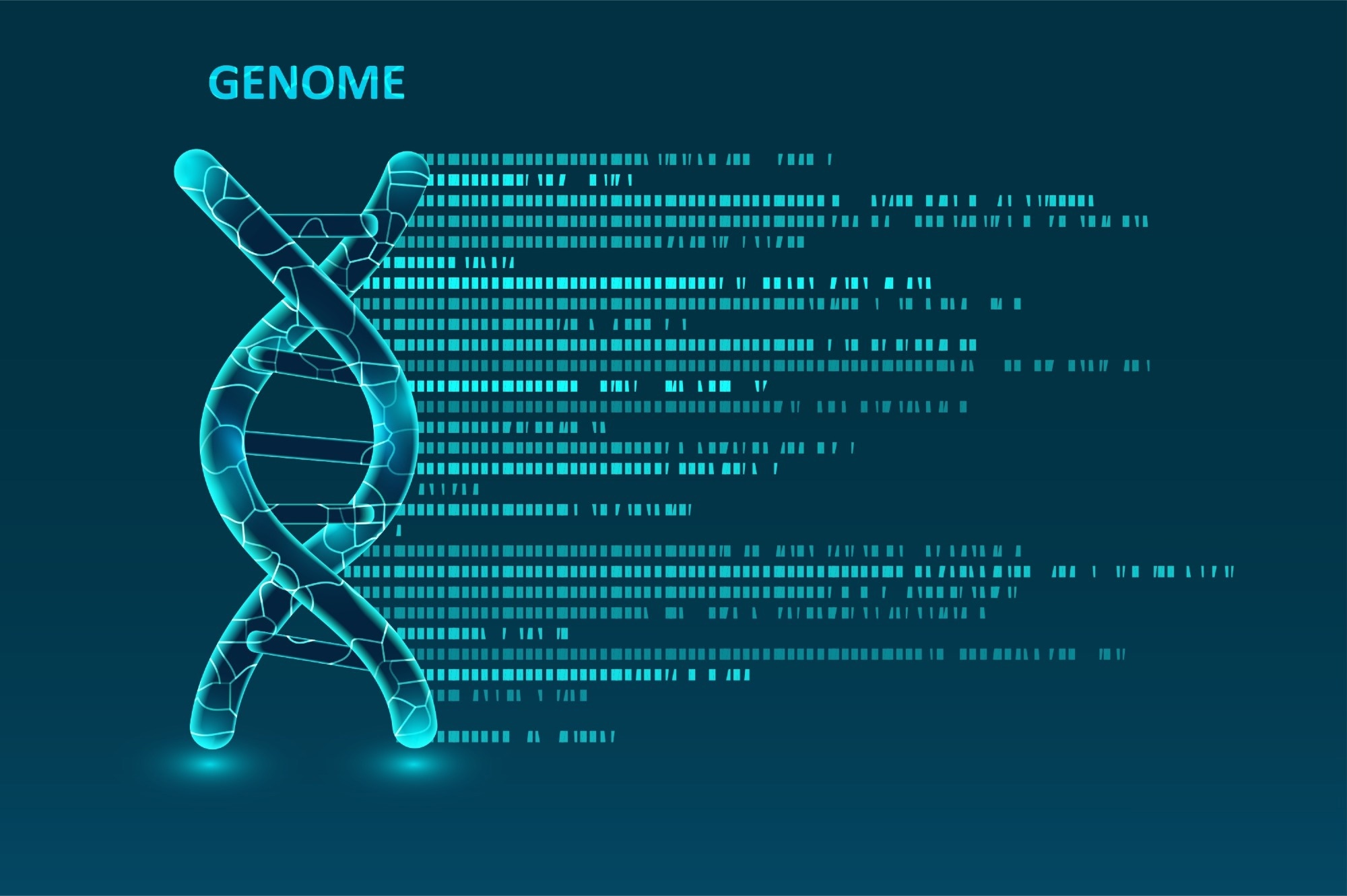
Image Credit: majcot/Shutterstock.com
With the 20th anniversary of the Human Genome Project and the 7th birthday of MGI both this April, how do you personally feel about how far the field of genomics has come? Where do you feel that this sector is heading?
I am really happy with how things have panned out. If we had not merged with MGI, the field would be in a totally different place than it is today. Yet, from the beginning, we have always had this feeling or hope that we could do this almost unlimited level of sequencing. There are so many genomes and other omics to sequence, and we are unlikely to stop improving even at $10 genome.
The whole field is unstoppable, and we will continue to do wonders, and I am certain that the true importance of massively paralleled sequencing or any large-scale, efficient sequencing is still underestimated.
The green energy revolution and electric vehicles are critical. But efficient sequencing, I think, will be recognized as one of the most important human technologies because it concerns our health. Our genome touches all aspects of our lives, including genetic needs for personalize parenting or personalized learning. The whole of medicine will be changed. Imagine having the ability to deeply, efficiently, and accurately measure your molecular health at an affordable price. When you combine that enormous data set with artificial intelligence, suddenly, we have predictions or preventions of disease and aging that are affordable to everybody.
About Dr. Radoje Drmanac
Dr. Radoje (Rade) Drmanac is the co-founder and has served as Chief Scientific Officer since June 2005 at Complete Genomics and Chief Scientific Officer since 2013 at MGI Tech. He invented and led development of massively parallel DNA sequencing using DNA nanoarrays, ligation-based DNA sequencing, and haplotyping by co-barcoded sequence reads.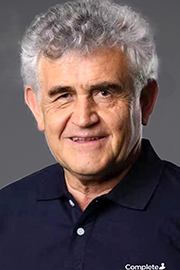
In 1994, Dr. Drmanac co-founded Hyseq Inc., a DNA array technology company that became Hyseq Pharmaceuticals, Inc. and later merged with Variagenics, Inc. to become Nuvelo, Inc., and served as its Senior Vice President of Research from 1994 to 1998 and as its Chief Scientific Officer from 1998 to 2001. Prior to that, Dr. Drmanac served as a group leader at Argonne National Laboratory from 1991 to 1994 as part of the Department of Energy’s Human Genome Project. He started his career at the Center for Genetic Engineering, later IMGGE, in Belgrade, Serbia. Dr. Drmanac received a B.S., M.S., and Ph.D. in Molecular Biology from the University of Belgrade.
*Unless otherwise informed, StandardMPS and CoolMPS sequencing reagents, and sequencers for use with such reagents are not available in Germany, Spain, UK, Sweden, Italy, Czech Republic, Switzerland and Hong Kong (CoolMPS is available in Hong Kong).
*Products are provided for Research Use Only. Not for use in diagnostic procedures (except as specifically noted)